All published articles of this journal are available on ScienceDirect.
A Level Playing Field for Comparing Air and Rail Travel Times
Abstract
Background:
Shifting travellers from air to rail can reduce environmental impacts and is an important European Union goal. Online travel planning applications allow travellers to easily compare air and rail transport choices, however, they may not accurately consider time that travellers spend at the airport or railway station since these depend on buffer times travellers use to protect against delays.
Methods:
This research investigated the actual time spent at airports and railway stations to analyse the accuracy of travel planning applications and helps improve the quality of travel time estimates. The research used a travel time recording application to determine the time spent by passengers at airports and railway stations. The data was collected for 312 trips. The research was supplemented by an extensive literature review of dwell times and multimodal travel planning applications.
Results:
The research found that travellers spent an average of 157 minutes at airports and 32 minutes at railway stations. Comparing these results to travel planning application, the information shows that the applications significantly underestimate time spent at airports and slightly underestimate time spent at railway stations. The use of unrealistic airport waiting times in travel planning applications distorts traveller perception in favour of air travel.
Conclusion:
Therefore, railway operators should support the development of improved travel planning applications that better consider waiting times. Improving these applications would be much more cost effective than infrastructure improvements designed to save a few minutes of travel time.
1. INTRODUCTION
A growing number of online travel planning applications provide multimodal door-to-door travel time estimates that travellers can use to compare travel times by air and rail transport. These travel time estimates depend on assumptions made for travellers' waiting time at airports and railway stations. These assumed waiting times have a significant impact on total travel time and therefore a traveller’s mode choice decision. The purpose of this research was to measure the different elements of door-to-door travel times by air and rail, and then compare these to data from several popular online travel planning applications to determine if these applications underestimate waiting time and assess how this could impact the mode choice decision between air and railway travel.
The next section presents an introduction to the effects of air and rail transport on climate change. It highlights the importance of competition between the two modes of transport. Given the high relevance of travel time for mode choice between these competing travel services, the last section of the introduction discusses the different segments of door-to-door travel time. Based on this, the second section presents the research method for determining real door-to-door travel times in air and rail transport. The empirical results are described in Section 3 and are discussed in Section 4, in particular, with regard to the strengths and weaknesses of the chosen research approach. The last section presents conclusions and recommendations.
1.1. Climate Change Impacts of Air and Railway Travel
Climate change is creating significant social, economic and environmental problems. Consequently, reducing climate change is a strategic priority for the European Union (EU) [1]. The European Commission’s goal is to reduce Greenhouse Gas (GHG) emissions, a major cause for climate change, to 80-95% by 2050 [2], with an intermediate target of a 40% reduction by 2030 (both relative to 1990 levels) [3].
In the transport sector, global GHG emissions are projected to double by 2050 without aggressive and sustained policy intervention according to the UN Intergovernmental Panel on Climate Change (IPCC) [4]. Consequently, the EC has set a goal for the transport sector to reduce its share of GHG emissions to 20% by 2030 as compared to 2008 levels, and to 70% by 2050 [5].
One of the key problems faced in reducing transport sector GHG emissions is the strong growth in air travel [6, 7]. Much of this growth has been stimulated by the rise of low-cost carriers. The number of flights in Europe increased by 80% between 1990 and 2014 and is forecast to grow by a further 45% between 2014 and 2035 [8]. Airbus forecasts an increase in intra-regional and domestic Revenue Passenger Kilometres (RPK) of 2.9% per year for the European market between 2016 and 2036 [9]. The International Air Transport Association (IATA) projects European passenger growth of 2.3% per year until 2036; this is 550 million passengers more than today and will result in over 1.5 billion passengers by 2036 [10].
The environmental impacts of air travel in Europe have increased significantly over the past 25 years. Direct emissions from aviation account for about 3% of the EU’s total GHG emissions. However, the impact of aviation on climate change is almost doubled when the full effects of radiative forcing are included [11]. If global aviation was a country, it would rank in the top 10 of emitters. Some studies forecast that global aviation will generate 22% of total GHG emissions by 2050 if the sector continues to fall behind efforts in other sectors [12].
Much research has been conducted on developing technical and operational measures such as CO2 standards for aircraft or sustainable biofuels, but studies show that these may be insufficient to significantly reduce aviation emissions in the next 20 years [8, 13, 14]. In fact, air traffic and GHG emission growth have outpaced aviation sector efficiency gains for decades due to the continuous increase in passengers [6].
Given the difficulties in reducing GHG emissions in the aviation sector, an important EU goal is to support a modal shift from air travel to rail [15]. Shifting air travel to rail reduces environmental impacts [16]. According to Eurostat, 47% of European air passengers travelled on intra-European routes in 2016, and other 17% on national routes [17]. Many of these trips could be shifted to electrically powered railways – especially to Europe’s High-Speed Rail (HSR) network which is expected to triple in length by 2030. The carbon footprint of electrically powered railways depends on the primary energy used to generate the electricity, but part of the energy is already generated with renewable sources today (e.g. 100% green electricity for German ICE fleet) and the European production of renewable energy is growing [18].
In order to achieve its GHG emissions target, the European Commission’s goal is that the majority of medium-distance passenger transport in 2050 would be by rail [4]. Unfortunately, current trends contradict these plans. Between 1995 and 2010, HSR travel grew significantly faster than air traffic (8.1% versus 3.1% per year), however, the trend has reversed since 2010. Since then, intra-European air traffic has increased by 3.8% per year in passenger-kilometres, almost twice as fast as HSR, which grew by 1.5% per year [19].
1.2. Short Review of Air-HSR-Competition
Many studies have explored the relationship between air transport and HSR [20]. Competition between HSR and air transport started in 1964 with the opening of the world’s first high-speed train service Tokaido Shinkansen between Tokyo and Osaka. In 1981, France opened the first European HSR line: the Train à Grande Vitesse (TGV) from Paris to Lyon. In contrast to the Shinkansen, which operated on dedicated tracks, the TGV was fully compatible with the existing railway network.
Germany (1991) and Spain (1992) followed France with their Intercity Express (ICE) and Alta Velocidad Española (AVE) trains. European HSR trains are capable of reaching speeds of over 200 km/h on upgraded conventional lines and of over 250 km/h on new lines designed specifically for high speeds [21, 22]. The improved quality and speed of HSR service enabled railways to return to competitiveness in the long-distance passenger transport market [23].
Research on the interaction between air transport and other modes initially focused on competition between modes; more recent research also considers modal complementarity [24]. Sun et al. synthesized and discussed recently published studies on competition between HSR and air transport [20]. Their meta-analysis finds that travel time is the most critical factor in determining the competitiveness between HSR and air transport. Janic developed one of the earliest models of travel time competition between the two modes, concluding that HSR can compete with air transport over a range of distances from 400 to over 2000 km [25]. Givoni found that the introduction of HSR on routes of around 300 km leads to an almost full withdrawal of airline service (e.g. between Frankfurt and Cologne or Brussels and Paris), while on routes of over 1000 km, HSR cannot seriously compete with air transport (e.g. between Tokyo and Fukuoka, 1070 km, the HSR share of the traffic is only 10%) [23]. In between 300 and 1000 km, HSR is most competitive against air transport [20, 23, 26]. Research from Steer Davies Gleave reduces this competitive range to between 175 and 800 km, where door-to-door travel times of HSR are shorter than air travel [27].
1.3. Traveller Scheduling Model
Given the importance of travel time on air–rail competition, it is necessary to develop realistic travel times. These travel times should consider the entire door-to-door travel chain. However, most published work focuses only on the in-vehicle time or selected elements of the entire door-to-door travel chain. In contrast, door-to-door travel is the complete chain of process steps including access from origin to the travel mode, waiting time, in-vehicle travel time, transfers, and egress from the mode to the final destination. Finally, it is critical to note that travellers often add buffer times to the minimum calculated time needed to perform these activities in order to account for delays or uncertainty. This is particularly true for air travel given the uncertainty of activities such as security control and the high penalty for missing a flight.
The in-vehicle travel times and transfer times can be easily obtained by travel planning and information applications, but it is much more difficult to determine the access to airport/railway station and egress from airport/railway station times (including time spent in activities at the airport/station). These two steps are considered in more detail below.
1.3.1. Access to Long-Distance Transport
The scheduling model of Noland and Small [28] has been widely accepted as a standard tool for analysing the effects of travel time variability [29]. The model assumes that travellers make a trade-off between being earlier or later than their preferred arrival time. Koster et al. extended the model to account for the specific concerns of air travellers. They found that the departure time from home chosen by air travellers depends strongly on the probability of missing a flight, and the corresponding expected cost [29].
The same cost calculations can be assumed for HSR travellers. However, the rail travellers plan against the background of different procedural conditions. (Fig. 1) illustrates the time versus cost structure for air (black) and rail travellers (red) using the approach from Koster et al. [29].
As shown in Fig. (1), there are three main differences between rail and air traveller’s cost structure for accessing the travel mode. These differences lead to structurally shorter process and waiting times at railway stations and have a decisive effect on the perceived time uncertainties and the resulting time buffers:
- Spatial structure: The main railway stations in most European cities are located closer to city centres and are better linked to regional transport networks than airports. This results in shorter minimum travel times to the station and reduced buffer times due to the availability of more travel alternatives in case of local service interruptions.
-
Process Structure: With a few exceptions (e.g. Eurostar services), European HSR systems are open-gated systems. There are no permanent security checks and luggage does not need to be checked-in separately. The process for boarding an HSR train generally consists of walking to the platform and stepping onto the train via many doors. The process for boarding a short/medium haul flight consists of a fixed sequence of successive handling processes (check-in, security control, walking to the gate, and boarding via one door). Furthermore, most people are much more familiar with railway stations and the train boarding process than they are with airports and the flight boarding process. This makes it particularly difficult to forecast the time
required for process steps such as baggage check-in and security checks. In summary, the minimum processing time and the buffer time to account for uncertainty are both higher for air travel than for railway travel.Fig. (1). Access cost functions of air and rail travellers (based on [29]). - Failure Costs: On average, the cost of an airline ticket is higher than an HSR ticket [30]. Furthermore, a much higher proportion of airline tickets are not easily changeable or refundable. These factors make the cost of missing a flight higher than missing an HSR trip. Finally, given the lower frequency of flights, even if an airline ticket can be used for a later flight, the risk of losing time due to a missed flight is noticeably higher than for a missed train since there is frequent railway service between many main destinations.
1.3.2. Egress from Long-Distance Transport
The process of exiting the long-distance travel vehicle (airplane, HSR) and leaving the airport or station also consists of several steps which differ significantly between the modes.
First, consider the time spent leaving the vehicle itself. On a typical high-speed train all the passengers on a single wagon leave via two doors (e.g., 74 passengers on an ICE 3 middle wagon leave through two doors). In contrast, all the passengers on an airplane generally must leave the aircraft via a single door (e.g., the approximately 160 passengers on a typical A320 at an airport using a passenger boarding bridge). When using a remote position with the following bus transfer, the average time to actually reach the terminal is even longer. This difference in time leaving the vehicle is exacerbated by the lower average capacity utilisation figures in long-distance rail travel: 53% (German rail) [31] vs. 83% in short-/medium-haul intra-EU air traffic [32, 33].
Second, many airline travellers also need to pick-up checked luggage, which adds to the time spent at the airport. In contrast, railway passengers keep their luggage with them through the trip. Finally, airports are much larger than railway stations which increases the travel time spent travelling from the gate to the baggage claim/exit, and then on to their connecting transport.
1.3.3. Research Question
As the analysis presented above shows, the process times for access and egress are structurally lower for HSR than air travel, and furthermore, HSR travel requires lower buffer times to ensure against delays in access processes (e.g., security). But how is this structural advantage for HSR reflected in mode choice decisions? Is it possible for travellers to obtain accurate door-to-door travel times from travel planning applications? How do these applications forecast these structural time differences and buffer times? How do buffer times correspond to real passenger behaviour? The research was carried out to help answer these questions.
2. RESEARCH METHODOLOGY
A three-step approach was used to address the research questions. The first step consisted of analysing the comparability of rail and air travel time information presented on digital travel information portals. The second step consisted of examining research on real airport/railway station access and egress times. The third step consisted of performing and analysing a survey of real door-to-door travel times for flights and railway trips in European long-distance travel.
2.1. Analysis of Digital Travel Information Systems
An increasing number of flight and train bookings are being made independently by travellers without the support of sales staff from travel providers. Information and booking are increasingly carried out via large online sales platforms, which are either intra- or multimodal. The research assessed how these information systems consider buffer times for long-distance travel.
The research started by identifying a set of travel planning application platforms that could be used by travellers making travel decisions for long distance travel. The core criterion for selecting these platforms was their multimodal design, thus, in principle, enabling them to compare travel times and prices between air and rail transport. The research started with platforms identified in the market analysis carried out for the European Commission’s DORA project [34]. These platforms were: GoEuro, Google Maps, Rome2Rio, Route Rank and Qixxit. This research added the platforms fromAtoB and Kayak. All other freely available platforms on the Internet did not meet the selection criteria.
The research examined each of the platforms to determine to what extent door-to-door travel time comparisons are actually possible and whether these comparisons are accurate. It identified the buffer times currently taken into account at railway stations and airports in the case of door-to-door information provided by the platforms. The analysis used the terminology and segmentation of the DATASET2050 project [35] to determine what time requirements were displayed (if any) for the following journey phases:
- Door-to-Kerb (D2K): Transfer from the starting address to the station/airport
- Kerb-to-Gate/Platform (K2G/K2P): journey segment from arrival at the point of access to the main means of travel (pre-travel dwell time)
- Gate/Platform to Gate/Platform (G2G/P2P): Main travel segment from boarding to alighting, includes the phases off-block, taxiing-out, take-off, route, landing, taxiing-in and on-block in air traffic.
- Gate/Platform-to-Kerb (G2K/P2K): Journey segment from gate/platform to connecting means of transport, also includes baggage reclaim and customs in air traffic (post-travel dwell time)
- Kerb-to-Door (K2D): Transfer from the station/ airport to the destination address
2.2. Examining Research on Real Airport/Railway Station Access and Egress Times
The authors carried out a literature review of previous research on airport/railway station access and egress time. This review focused especially on dwell times, the length of stay in airports or stations. Given the very small amount of dwell time data in published academic literature, the researchers also used data from airport and railway station operators in the analysis. Operators have a high incentive for collecting this dwell time because it is an important indicator for marketing sales and advertising space.
2.3. Survey of Real Door-to-Door Travel Times in European Long-Distance Traffic
The main part of this research was collecting and analysing door-to-door travel times for medium to long-distance trips by air and rail. Data were collected by an existing panel organised by an independent quality and market research institute. The panel currently consists of more than 4,500 active participants in private households throughout Germany. As part of this research, the panel participants were provided with a mobile telephone application specifically developed for this project to measure travel times by travel segment. This application was designed as a hybrid mobile app with the web framework Ionic. Hybrid mobile apps combine the advantages of native and web apps. By providing platform-specific libraries, which allow access to the hardware and software components, they run on different operating systems (e.g. Android, iOS, Windows). The user interface was created with HTML and SASS/CSS, the logic was programmed with JavaScript. The use of the app was voluntary and was supported by an incentive bonus system, which is also used with other surveys.
The application provided participants with a simple and intuitive interface to delimit five stages of their journey in time while the device simultaneously logged their location via GPS. Participants simply pressed the app button at the following points of their trip:
- Leave starting address
- Arrival at the station/airport
- Departure
- Arrival at destination station/landing at the destination airport
- Leaving station/airport
- Arrival at the destination address
The application then automatically registered the time and place. Travellers were able to manually correct time-related data and add comments, but the GPS-data was not accessible. In addition, the participant entered manually whether the trip was a private or business trip. (Fig. 2) presents application screenshots for entering data on a private rail trip and a visualisation of results for the last two travel stages.
A total of 312 trips were recorded in the period from March to October 2017. These included 74 air trips and 238 train trips from and to seven German cities: Berlin, Cologne, Frankfurt, Hamburg, Hannover, Leipzig, Munich. The duration data for the following five travel stages were generated from the raw data of the app-supported manual tracking:
- Transfer time to airport/station
- Time between arrival at the airport/station and start of the flight/rail travel (dwell time 1)
- Flight time / rail travel time
- Time between arrival at the destination airport/station and leaving the airport/station to the final destination address (dwell time 2)
- Transfer to destination
The analysis calculated average stage travel times for business trips, private trips and all trips.
Given the resources available, the aim was not to conduct an extensive survey but rather to perform an initial study which could be used as a basis for a more far-reaching subsequent study.
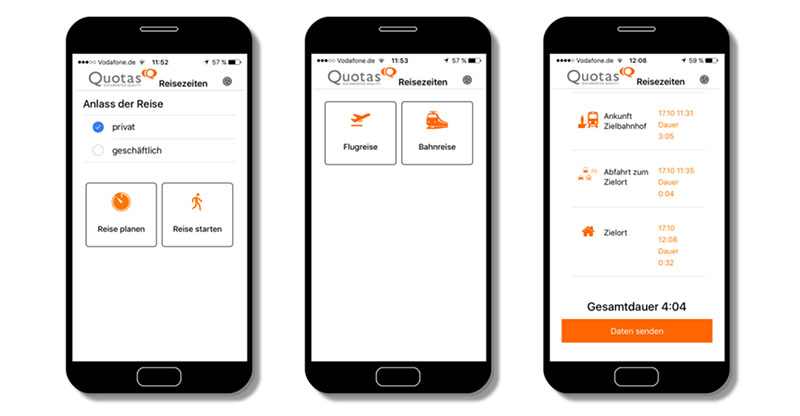
3. RESULTS
The results show that most of the travel planning applications examined in the research do not provide full door-to-door travel times for both air and rail trips. Furthermore, those applications that do provide this information clearly underestimate the length of time spent at the access and egress points for long-distance transportation (i.e., at airports and railway stations). An important reason is that lack of sufficient travel time data for the access and egress stages of the journey. The travel survey application data collected in this research showed that air travellers reach the airport on average just under two hours before departure, showing that waiting time at the airport is a significant component of total travel time. These results are discussed in the following sections.
3.1. Travel Information Portals Obscure True Travel Times
Table 1 summarises the type of travel time information provided by the seven travel planning applications examined in this research.
In terms of comparing multimodal trips (i.e., trips that include local access to the railway station or airport) only RouteRank and Rome2Rio provide users with total travel times for both air and rail journeys. RouteRank provides public transport stop to public transport stop travel times for air and door-to-door travel times for rail journeys, while Rome2Rio provides door-to-door travel times for both air and rail journeys. Google Maps provides door-to-door travel times for rail trips but does not include local access time in its travel times for air. All the other applications examined use public transport station to public transport station data for rail journeys and airport to airport data for flight alternatives.
Only two of the applications examined consider waiting time at airports in their travel time estimates. RouteRank provides for an airport arrival at least 90 minutes before departure. This is consistent with official recommendations for intra-European flights. Rome2Rio, on the other hand, provides for an airport arrival only 60 minutes before departure, even at large international hubs such as Frankfurt.
RouteRank and Rome2Rio are also the only two applications that consider time from aircraft arrival at the gate to departure from the airport in their air travel time estimates. RouteRank adds a 60 minute transfer time between landing and onward journey. Rome2Rio includes a transfer time of 10 minutes.
For rail trips all the applications except Kayak include transfer times to/from the station entrance and platform based on minimum walking times, but do not include any buffer for potential delays in reaching the station, nor do they consider orientation time (e.g., the time needed to determine the correct platform).
The analysis clearly shows that railway travel is placed at a disadvantage in all the travel planning applications. First, the applications provide flight only (off block/on block) travel times for air travel, but total travel times including local access/egress for railway travel. Second, the applications that take airport waiting times into consideration appear to underestimate these times. Finally, all the applications appear to underestimate railway station waiting times.
3.2. Poor Data on Passenger Dwell Times at Access and Egress Points
While it seems likely that the two applications that include airport waiting time underestimate this time, there is very little empirical data on airport waiting time in the transport research literature. Small sample surveys conducted in Australia show an average airport stay of 111 or 113 minutes before departure [36, 37].
fromAtoB | GoEuro | Google Maps | Kayak | Rome2Rio | RouteRank | Qixxit | |
---|---|---|---|---|---|---|---|
D2D search | no | no | yes | no | yes | yes | no |
Intermodal travel-chains | no | no | no | no | yes | yes | no |
AIR – Display of Travel Times Per Journey Segment | |||||||
D2D travel time | no | no | no | no | yes | (yes) | no |
D2K segment | no | no | no | no | yes | PT stop to Kerb | no |
K2G segment | no | no | no | no | Minimum 60 min | Minimum 90 min | no |
G2G segment | yes | yes | yes | yes | yes | yes | yes |
G2K segment | no | no | no | no | Minimum 10 min | Minimum 60 min | no |
K2D segment | no | no | no | no | yes | Kerb to PT-stop | no |
RAIL – Display of Travel Times Per Journey Segment | |||||||
D2D travel time | no | no | yes | no | yes | yes | no |
D2K segment | PT-stop to Kerb | PT-stop to kerb | yes | no | yes | yes | PT-stop to Kerb |
K2P segment | Transfer time without buffer | Transfer time without buffer | Transfer time without buffer | no | Transfer time without buffer | Transfer time without buffer | Transfer time without buffer |
P2P segment | yes | yes | yes | yes | yes | yes | yes |
P2K segment | Transfer time without buffer | Transfer time without buffer | Transfer time without buffer | no | Transfer time without buffer | Transfer time without buffer | Transfer time without buffer |
K2D segment | Kerb to PT-stop | Kerb to PT-stop | yes | no | yes | yes | Kerb to PT-stop |
Transfer to Airport |
Dwell Time at the Airport |
Flight Time | Dwell Time at the Airport |
Transfer to Destination |
Total Travel Time | |
---|---|---|---|---|---|---|
All trips | 57 | 118 | 143 | 39 | 61 | 417 |
Private trips | 58 | 123 | 154 | 43 | 64 | 442 |
Business trips | 55 | 101 | 113 | 25 | 56 | 350 |
Transfer to Station |
Dwell Time at the Station |
Rail travel time | Dwell time at the Station |
Transfer to Destination |
Total travel time | |
---|---|---|---|---|---|---|
All trips | 24 | 20 | 198 | 12 | 21 | 275 |
Private trips | 23 | 20 | 211 | 12 | 20 | 286 |
Business trips | 24 | 20 | 163 | 12 | 21 | 240 |
On the other hand, many airports regularly survey how long departing air travellers stay at the airport because this time has a strong impact on income. These surveys are generally based on questionnaires of departing passengers and not the result of accurate measurements [38]. The published data varies considerably in scope and differentiation. For example, Amsterdam Schiphol expects local boarding passengers to spend 147 minutes at the airport [39]. Zurich Airport determined that 54% of local passengers spend more than 90 minutes at the airport [40]. The Airport Commercial Revenue Study (ACRS), the travel retail industry’s leading study benchmarking the commercial performance of world airports, finds that international intra-EU travellers spend an average of approximately 99 minutes and domestic travellers in Europe spend approximately 93 minutes at the airport [41, 42].
The data situation for long-distance rail transport is significantly worse. There are no pan-European or pan-national studies on the length of stay at the origin and destination stations. Only studies for selected stations such as Nanjing South Railway Station [43] or Linz [44] are documented. However, neither of these studies made a distinction between long-distance and local travellers.
Most current research (e.g [45, 46].) references the work of Cokasova from 2003 which determined the stay durations at the origin and destination of long-distance transportation for both rail and air travel [47]. The research determined an average stay duration of 60 minutes at the origin airport and 20 minutes at the destination airport. For rail traffic, it determined a 10-minute stay at the origin station and assumed the time required for transferring to local transport at the destination station to be 0 minutes.
However, the ACRS findings and more recent transport modelling indicates that Cokasova’s time estimates probably significantly underestimate actual waiting times. The DATASET2050 project of the Horizon 2020 research programme modelled door-to-door travel times for air travel between the 200 major European airports. The average stay at the origin airport before departure was 114 minutes. The model also showed that it takes the traveller 31 minutes after arrival to get from the aircraft to the means of local access transport [35].
3.3. Travel Time Analysis Confirms the Importance of the Dwell Time Factor
The travel time survey application data collected as part of this study confirms the assumption that actual travel behaviour leads to significantly longer buffer times for air transport than previous assumptions in the scientific literature or current travel information systems.
As shown in Table 2, for all trips the average stay at the airport before departure was just under 118 minutes and the average time spent after landing was 39 minutes. These values remain relatively stable if the sample is reduced to trips with flight times of less than three hours (113/41 minutes) or even less than 90 minutes (112/42 minutes). Business travellers have shorter stays, but these are still well above Cokasova's assumptions and the calculation bases in the travel planning information systems. The biggest time difference between business and private trips is at the destination airport and is likely due to business travellers carrying only hand luggage and choosing seats in the front of the aircraft.
In contrast, there are almost no differences in the length of stay for business and private travellers at railway stations. As shown in Table 3, passengers spend an average of about 20 minutes before, and 12 minutes after the train journey in the station. These empirical results also clearly exceed the frequently quoted assumptions of Cokasova. However, the differences from assumptions in the travel planning information systems are significantly less than for air travel.
4. DISCUSSION
This study is the first worldwide to present an app-supported determination of travel segment duration for all segments of door-to-door long-distance journeys using the same methodology for rail and air travel. While travel segment durations were determined in previous studies, this was usually done via ex-post surveys rather than using real-time travel-accompanying surveys supported by software. This helped improve data quality by reducing errors typical of retrospective interviews such as false perceptions of time or statements of social desirability. Against this background, the quality of the survey results is rated as very high compared to previous time recordings of travel segments.
However, the meaningfulness of the average dwell time values identified in this study is limited by the small sample size and the limitation to analyses of flights within or from/to Europe. The measured dwell times show a high standard deviation. This suggests that individual user travel experience has a strong influence on travel planning and the integration of time buffers of different lengths into travel planning. In air travel, the transport of baggage, in particular, is likely to have a considerable effect on the time spent at the airport. However, since the baggage carrying feature has not been recorded separately, it is unfortunately not possible to verify the corresponding theses.
The comparison of the online travel planning applications with regard to their included travel segments and time requirement assumptions has not yet been carried out in this form in any publication. Here the research shows the clear differences in travel time calculation methods. However, the development of these online travel planning tools is highly dynamic. The research therefore only provides a snapshot of the current situation. Moreover, the selection of travel planning tools only allows statements for the European market. However, the lack of airport-specific differentiation and individual adaptability of the Kerb-to-Gate and Gate-to-Kerb segments in all tools evaluated shows the continuing underestimation of the importance of these travel stages and makes it difficult for these tools to present a fair comparison of rail versus air travel times for long distance journeys.
CONCLUSION
The study results show that current travel planning applications distort actual door-to-door travel times. More specifically, they significantly underestimate travel times by air for two reasons. First, they do not include realistic waiting times at the origin and destination airports. Second, most applications do not include local access and egress times to airports but do include them for rail.
The study points to several areas for more research. First, the finding that waiting times at airports are underestimated is based on relatively small sample size and therefore a more extensive data collection and analysis should be performed. Future research should also consider to what degree passengers perceive time spent waiting at the airport or railway station positively. It is possible that people use the time constructively by shopping, eating or working. Another topic to consider is how the size of airports and train stations impacts the accuracy of travel time information. Second, the evaluation of travel planning applications shows a very strong need for improving the comparability of multimodal trips.
High-speed rail operators should be particularly interested in making the door-to-door travel time differences between air and rail travel clearly visible to travellers since these differences help justify the major investments needed for high-speed rail. In this respect, high-speed rail operators should support more research on time spent at airports and railway stations, and the development of improved multimodal travel time comparison portals that better capture door-to-door travel times.
Studies have repeatedly confirmed the dominant importance of travel time for the choice of transport mode. In view of the fact that process times at airports have been significantly underestimated in all the internet-based multimodal comparison portals evaluated in this research, there is considerable potential for growth in railway trips by providing users with more accurate door-to-door travel time comparisons. These corrections should lead to an improvement in the relative competitiveness of rail and would help justify the large investments made in European railway infrastructure.
CONSENT FOR PUBLICATION
Not applicable.
AVAILABILITY OF DATA AND MATERIALS
The data supporting the findings of the article is available in the figshare repository at https://figshare.com/, reference number 6400832 (https://doi.org/10.6084/m9.figshare.6400832).
FUNDING
Our research project was funded by the International Union of Railways (UIC)
CONFLICT OF INTEREST
The authors confirm that this article content has no conflict of interest.
ACKNOWLEDGEMENTS
The submission builds on the results of the project “Real Travel Times Scoping Survey 2017. Door-to-Door analysis for long-distance rail and air travel” that was supported by the International Union of Railways (UIC). The authors express their thanks to the UIC Intercity & Highspeed Committee for all the support. Special thanks to our colleague Andrew Nash for his valuable feedback and suggestions on previous drafts of the paper.