All published articles of this journal are available on ScienceDirect.
Comparative Study of Mechanistic-empirical Structural Design Methods Applied to Sustainable Asphalt Mixtures
Abstract
Introduction/ Background
Nowadays, there is a growing interest in achieving sustainable alternatives to conventional flexible pavements. The incorporation of recycled materials into asphalt mixtures has become a departure point. This study focuses on developing sustainable asphalt mixtures incorporating different residues to find a material that performs well on both technical and environmental grounds.
Materials and Methods
Mixtures with recycled materials and others are compared on a pavement structure. A mechanistic-empirical pavement design method is used to carry out analyses in different conditions. A Life Cycle Assessment is conducted to consider the balance of positive and negative environmental aspects of each mixture and pavement structure.
Results
The results indicate that all mixtures with sustainable features have a longer life span and an enhanced performance. They also incur higher impacts per tonne produced. However, the “avoided impact” is considered in their favour.
Discussion
Mixtures with enhanced mechanical properties are key to achieving long-term environmental benefits. The usage of local materials is also essential.
Conclusion
Proving that unconventional mixtures have a satisfactory performance requires innovative, properly calibrated models and a thorough material characterization. Accurately reflecting on their in-service behaviour is key to evaluating the environmental benefits.
1. INTRODUCTION
Sustainability is a concept that refers to the possibility of conducting an activity sustainably over time, i.e., without harming the environment or depleting its resources, ensuring they remain available for future generations. The challenge is to incorporate sustainability into different areas of economic and social development [1]. In particular, this study aims to apply these ideas to the design of flexible pavements and relate them to the concept of Sustainable Pavements, thus contributing to completing another stage of the PaSos Project that the Road Laboratory of the IMAE - UNR has been conducting for more than two decades [2].
This study compares the expected service life of different flexible pavement structures in which sustainable asphalt mixtures are included. Structural behavior is evaluated using a mechanistic-empirical design method, both in initial and incremental conditions, based on studies conducted by Giovanon et al. (2013, 2022). [3, 4].
To achieve environmentally friendly asphalt mixtures, various approaches are considered:
- Incorporating recycled waste materials to reduce their accumulation in landfills.
- Reducing energy consumption and emissions using warm asphalt mixtures (lower production temperature).
- Using asphalt mixtures with greater durability or better mechanical properties, as they require less maintenance and have a longer service life.
For mixtures with recycled materials, the study considers including recycled plastics from silo-bags (SB), which are made of Low-Density Polyethylene (LDPE), and Phytosanitary Drums (PD), which are constituted by High-Density Polyethylene (HDPE), and rubber from End of Life Tires (ELTs). The selection is based on the fact that significant volumes of silo-bag and phytosanitary drums waste are generated in Argentina, as they are widely used in the country for agricultural purposes, and these materials are suitable to be added into asphalt mixtures due to the physicochemical compatibility between the asphalt binder and the polyethylene [5-7]. Additionally, there is a significant increase in goods transportation by trucks in the country, leading to the generation of significant quantities of ELTs. The addition of rubber from ELTs to asphalt mixtures is considered to improve flexibility, durability, aging resistance, and thermal susceptibility [8, 9].
On the other hand, Warm Mixtures (WM) are part of a relatively new, cost-effective, and environmentally friendly technology where the binder is an asphalt emulsion. These asphalt mixtures are produced using a combination of aggregates heated to approximately 120ºC and a certain amount of bituminous emulsion heated to a temperature of around 60ºC, which results in a mixing and compaction temperature between 80 and 90 °C [10-12].
Among the more durable asphalt mixtures, mixtures with Polymer-Modified Asphalt (PMA) and Highly Polymer-Modified Asphalt (HIMA) [13] are analyzed. All asphalt mixtures presented so far will be compared to an asphalt mixture made with conventional CA30 binder to be used as a control mixture.
The structural comparison is carried out by analyzing a pavement structure where only the material of the base layer varies, using the previously presented asphalt mixtures and keeping the rest of the layers invariable.
The BackViDe software [7] developed at IMAE (Instituto de Mecánica Aplicada y Estructuras- Institute of Applied Mechanics and Structures) is used for structural design. This software includes calibrated formulations for their application in Argentina. In the initial stage, the “VIDA” module of the software is used, which presents the analysis in initial conditions. Then, the “DETERIORO” component of the software is employed, which implements an incremental model to describe the evolution of the structure in service.
2. MATERIALS AND METHODS
To proceed with the design of the proposed structures, it is necessary to know the different parameters and functions that describe the behaviour of the component materials, particularly asphalt mixtures. Their physical and volumetric properties are described as follows. Their dynamic behaviour is analyzed based on the master curves of dynamic modulus. Also, their performance regarding fatigue and permanent deformation (rutting) is presented.
2.1. Asphalt Mixtures Description
The asphalt mixtures considered in this study are:
2.1.1. SBDW: Silo-Bags Dry-Way
Dense-graded hot mix asphalt with 2% (in weight, over the total mix) of pelletized LDPE, incorporated via a dry process. The asphalt binder is CA20-type [14]. This mix was designed and characterized in previous studies [6].
2.1.2. SBWW: Silo-Bags Wet-Way
Dense-graded hot mix asphalt with 3% (in weight, over the total binder – i.e., 0.15% over the total mix) of pelletized LDPE, incorporated via a wet process. The asphalt binder is CA20-type. This mix was designed and characterized in previous studies [6].
2.1.3. PDDW: Phytosanitary Drums Dry-Way
Dense-graded hot mix asphalt with 2% (in weight, over the total mix) of pelletized HDPE, incorporated via a dry process. The asphalt binder is CA20-type. This mix was designed and characterized in previous studies [6].
2.1.4. PDDW: Phytosanitary Drums Wet-Way
Dense-graded hot mix asphalt with 3% (in weight, over the total binder – i.e. 0.15% over the total mix) of pelletized HDPE, incorporated via a wet process. The asphalt binder is CA20-type. This mix was designed and characterized in previous studies [6].
2.1.5. 3M: 3% Micronized Plastic
Dense-graded hot mix asphalt with 3% (in weight, over the total mix) of micronized polyethylene pellets, incorporated via a dry process [15].
2.1.6. ELT: Rubber from End-Of-Life Tyres
Dense-graded hot mix asphalt with 10% (in weight, over the total binder – i.e. 0.47% over the total mix) of rubber powder [12].
The mentioned residues are illustrated in Fig. (1).
2.1.7. WMA: Warm-Mix Asphalt
Semi-dense graded warm mix asphalt elaborated with super-stable cationic bituminous emulsion as a binder (being its base binder CA30-type bitumen). For the production of this mixture, the aggregates are heated at approximately 120 °C and the bituminous emulsion at 60°C [14].
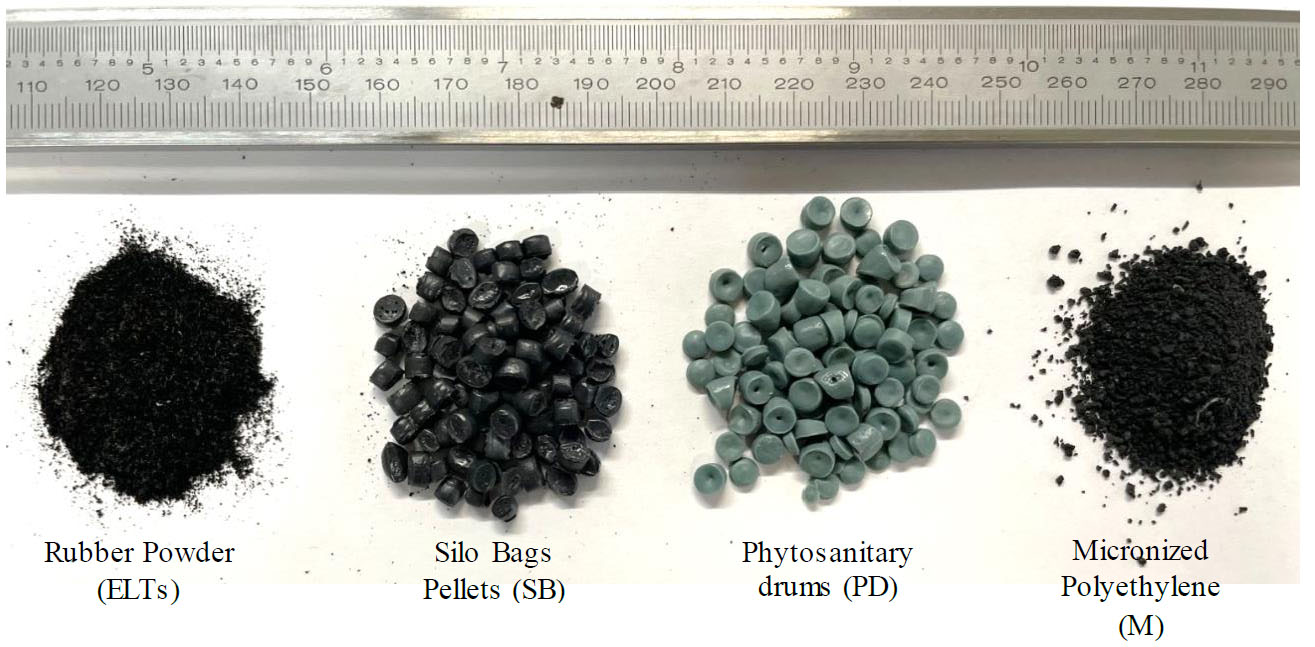
Residues incorporated into the asphalt mixtures.
2.1.8. PMA: Polymer Modified Asphalt
Dense-graded hot mix asphalt using AM3-type bitumen (3-4% SBS) as a binder.
2.2. Characterization
Table 1 describes the main physical-mechanical characteristics of the studied mixtures, including their volumetric properties.
Concerning the analysis of the mixtures' behaviour, Fig. (2) demonstratesthe master curves of dynamic modulus as a function of temperature for a frequency of 10 Hz. (UNE-EN-12697-26 Annex F).
To apply a mechanistic-empirical method like Back ViDe, it is necessary to know the failure criteria of the structural materials. These criteria are obtained from laboratory tests and are adjusted by a shift factor that considers their real in situ behaviour [7].
Fig. (3) shows the fatigue cracking failure criteria of the different asphalt mixtures evaluated, derived from Four-Point Bending Beam Tests (4PBT) (UNE-EN-12697- 24).
No. | Description | Bulk Density (kg/dm3) |
Max. Theor. Density (kg/dm3) |
Voids (%) | VMA (%) | VFA (%) | Binder Content (%weight*) | Recycled Material Content (%weight*) |
---|---|---|---|---|---|---|---|---|
1 | SBDW | 2,325 | 2,443 | 4,8 | 16,4 | 70,78 | 5,0 | 2,00 |
2 | SBWW | 2,447 | 2,540 | 3,7 | 15,9 | 76,78 | 5,0 | 0,15 |
3 | PDDW | 2,300 | 2,465 | 6,7 | 18,2 | 63,19 | 5,0 | 2,00 |
4 | PDWW | 2,426 | 2,557 | 5,1 | 17,2 | 70,40 | 5,0 | 0,15 |
5 | 3M | 2,340 | 2,450 | 4,0 | 15,0 | 73,33 | 4,7 | 3,00 |
6 | ELT | 2,446 | 2,608 | 6,1 | 17,6 | 65,33 | 4,7 | 0,47 |
7 | WMA | 2,428 | 2,550 | 4,8 | 16,9 | 71,66 | 5,0 | - |
8 | PMA | 2,470 | 2,625 | 5,9 | 16,7 | 64,67 | 4,4 | - |
9 | HIMA | 2,420 | 2,497 | 3,1 | 15,9 | 80,50 | 5,3 | - |
10 | HMA | 2,380 | 2,487 | 4,3 | 15,7 | 72,61 | 4,8 | - |
Note: *over the total mix.
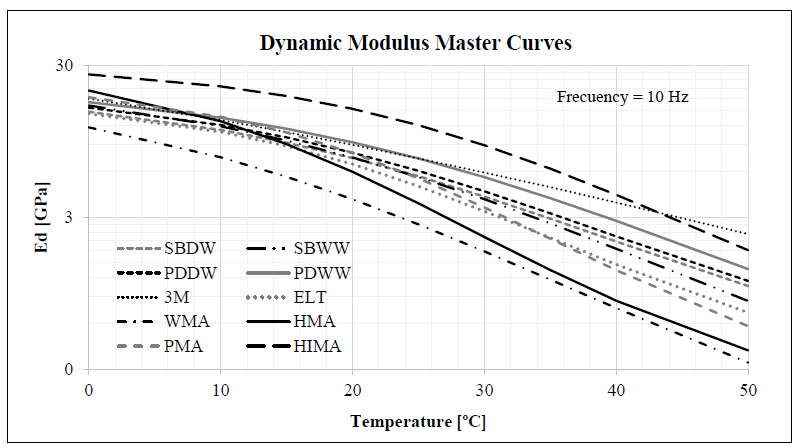
Dynamic modulus (Ed) master curves.
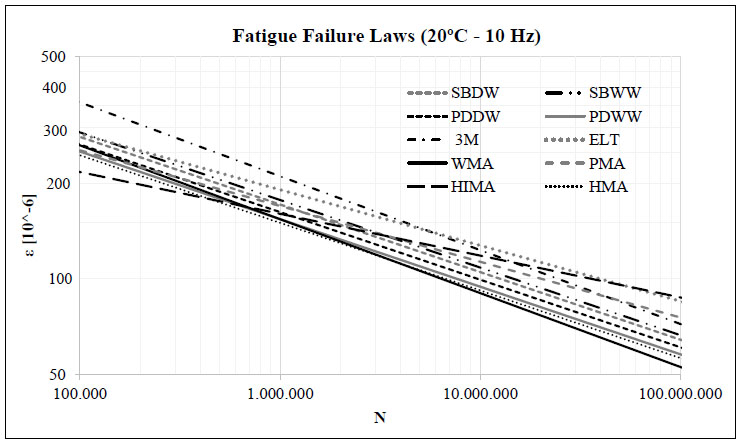
Fatigue behaviour of asphalt mixtures.
Fig. (4) displays the rutting evolution in the Wheel Tracking Test (WTT) (UNE-EN-12697-22) for the different mixtures studied as a function of the number of load applications.
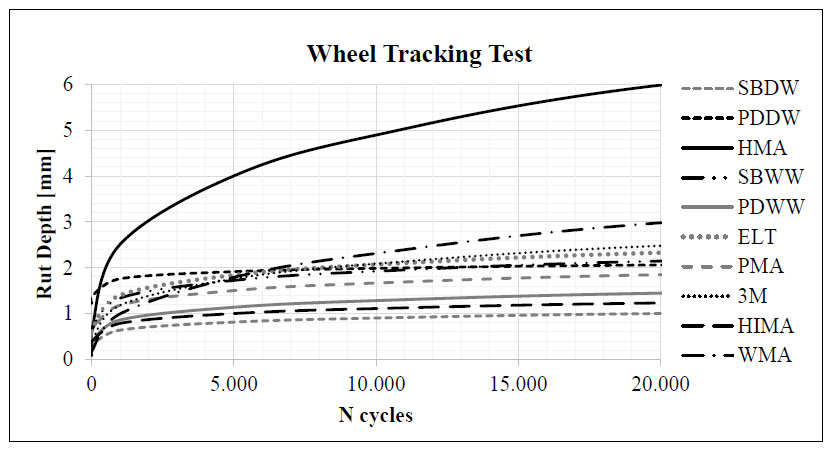
Rutting behaviour of asphalt mixtures.
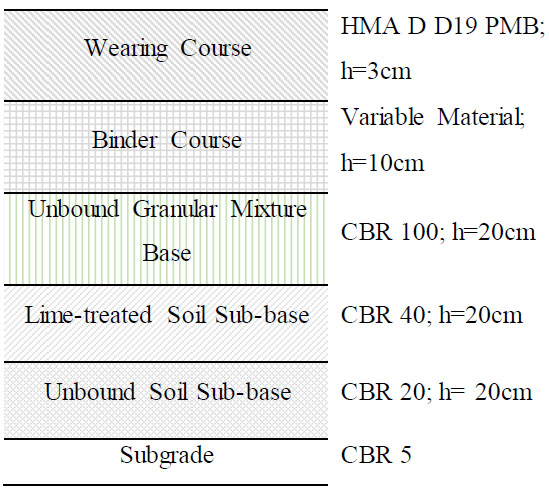
Typical structure schematics.
2.3. Structural Analysis
2.3.1. Structural Design of Flexible Pavements
Fig. (5) illustrates the pavement structure under study and its characteristics. Ten different structures are obtained from varying the material components of the asphalt base (among those of Table 1). The asphalt materials (base and wearing course) are characterized according to their dynamic modulus master curve and their behaviour under fatigue and rutting, as mentioned earlier. The structural behaviour of the unbound materials, constituting the lower layers, is defined according to the constitutive equation of the material, resulting in a nonlinear elastic analysis in which the Resilient Modulus (MR) is defined based on the stresses to which it is subjected at each point. Additionally, as a brief description of each layer's characteristics, the associated CBR is indicated.
2.3.2. Structural Design Methods
To proceed with the structural behaviour evaluation of the different structures, the Mechanistic-Empirical Design Method BackViDe is applied, conducting a structural analysis for initial and incremental conditions (deterioration).
2.3.2.1. BackViDe Initial Conditions
Firstly, an empirical-mechanistic method based on initial conditions is used, such as the “VIDA” component of the BackViDe software. The analysis under initial conditions allows us to determine the structure’s service life in terms of years or ESALs, using a fixed dynamic module, Ed, at 10 Hz and in correspondence with the mean temperature of the asphalt mixture in an average Argentinian location. The software considers a 30% cracked surface as a fatigue failure criterion and a 12 mm rut depth as a criterion for cumulative deformation. It also establishes that failure in the bound layers occurs due to cracking (repetition of Ɛt, which is a specific tensile strain at the bottom of the asphalt base), and in the unbound layers, failure occurs due to permanent deformation (accumulation of Ɛp, which is a specific compression strain on the subgrade).
2.3.2.2. BackViDe Incremental Conditions
The evaluation under Incremental Conditions is carried out through the “DETERIORO” module of the BackViDe software. The analysis under incremental conditions allows us to understand the evolution of the damage the pavement will sustain over time in terms of pavement distress and functional performance (cracking, rutting, and roughness). This methodology takes into account the variation of the asphalt layers' modulus based on temperature. Climate data from the typical location of the road and the estimated traffic evolution are used for this purpose.
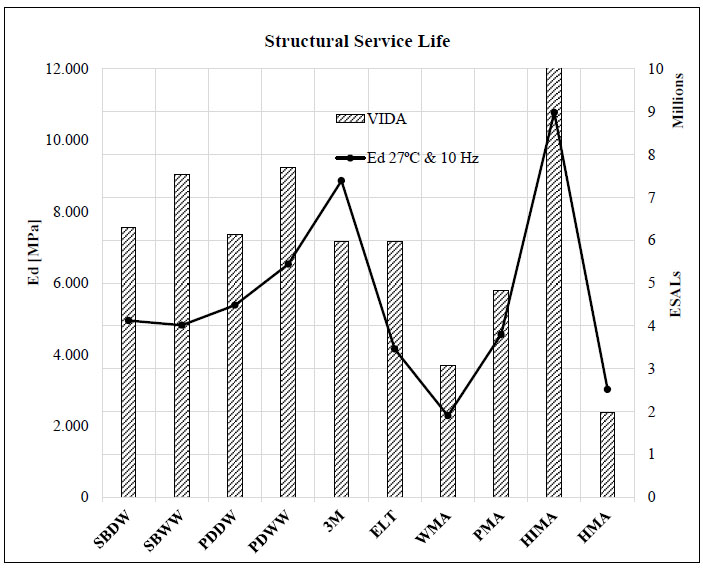
Module of binder course layer and Service Life of the studied structures according to initial conditions modelization.
2.3.3. Solicitations
To complement the study, traffic demands are defined as Equivalent Single-Axle Loads (ESALs) with a destructive effect of 80 kN. Therefore, a traffic volume of 1 million ESALs is considered for the initial year, with a projected annual growth rate of 3.0%. The average temperature of the asphalt mixture considered for the analysis under initial conditions is 27ºC since the structure will be located in a typical region of Argentina.
3. RESULTS AND DISCUSSION
3.1. Initial Conditions Model: VIDA
After defining each of the involved layers and processing the different structures using the VIDA module of BackViDe, the results presented in Fig. (6) are obtained, showing the service life and the adopted Ed. It is highlighted that all structures reach the end of their service life due to fatigue failure of the 10 cm-thick asphalt base..
The results indicate that the incorporation of recycled plastics, as well as rubber, has improved the characteristics of asphalt mixes compared to those of the control mix. Additionally, it is observed that all mixes with sustainable features have a longer service life than the HMA. The differential performance of the HIMA mix is also evident, surpassing 30 million ESALs, which represents a service life well above 20 years.
3.2. Incremental Conditions Model: DETERIORO
Firstly, surface rutting is analyzed after processing the structures through the DETERIORO module in BackViDe. Though the contribution of all layers is considered, the permanent deformation occurring at the subgrade level is the most significant one. In Fig. (7), the evolution of rutting and the adopted failure criterion are shown, and the intersection of both defines the service life of each structure.
Secondly, the progression of surface cracking (“bottom-up” type) is evaluated. Fig. (8) shows the adopted failure criterion and the cracked surface percentage, whose growth is caused by crack propagation. Notably, Fig. (8) does not include the HIMA mix because, due to its characteristics, cracking initiation occurs beyond the 30 years studied.
The results indicate that all studied structures fail due to rutting. As expected, mixes with shorter service lives are HMA and WMA, showing similar performance as they both have the same base binder. The other sustainable mixes evidently improve their performance, attaining service lives greater than that of the control mix. Mixes with recycled plastics have a life increase between 90% and 150%, approximately doubling their lifespan. A similar case occurs with PMA. The mix with ELTs shows a 175% increase in life and 3M, a 250%. Finally, the HIMA mix has a five-time-longer lifespan.
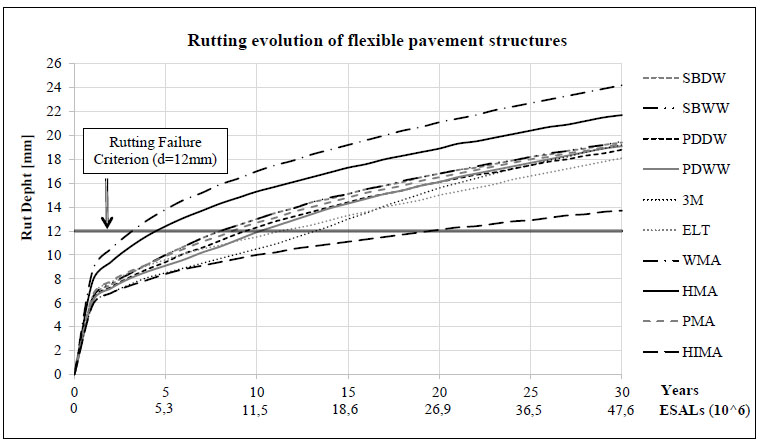
Evolution of rutting and rutting-related life expenctancy, obtained by means of incremental conditions modelization.
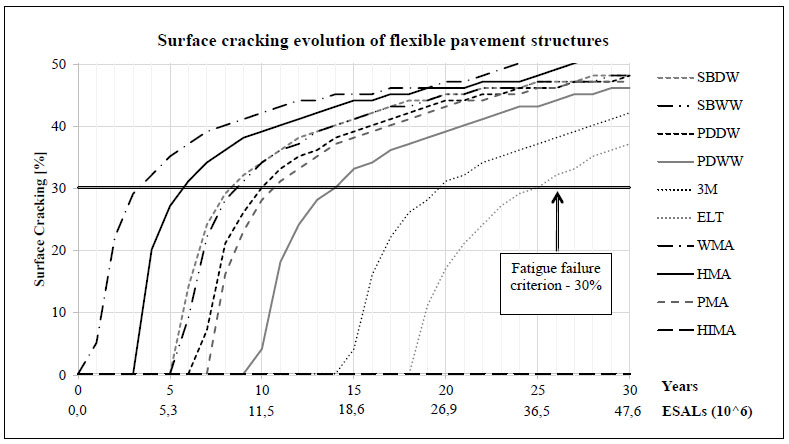
The evolution of surface cracking and fatigue-related life expenctancy is obtained by means of incremental conditions modelization.
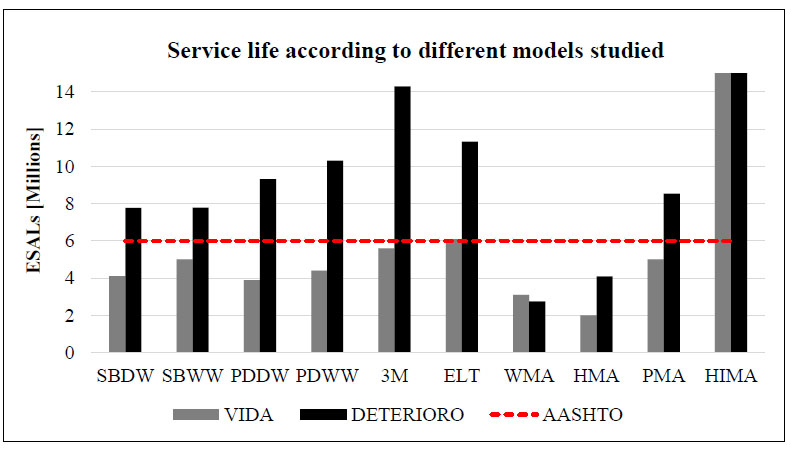
Comparison of service life obtained by means of different models and methods considering a 10 cm-thick asphalt base.
In Fig. (9), the results obtained from the initial (VIDA) and incremental condition (DETERIORO) analyses are compared. Additionally, just for comparative purposes, the number of ESALs to failure that is obtained by the AASHTO 93’ method, still used in Argentina, is indicated. As the thickness of each layer remains constant, the expected life of every structure differs. In subsequent studies, pavement structures designed for a given lifespan of 15 years will be processed, and the differences in the thicknesses of the base material required to achieve this will be compared.
3.3. Environmental Considerations
Having analyzed the behaviour of sustainable mixes through different models and concluded that they have a longer lifespan and better performance than conventional mixes, it is considered that all of them are suitable to be included in a flexible pavement structure. However, this is not sufficient, and therefore the next step is to evaluate the environmental benefits of each by using a Life Cycle Assessment (LCA) (ISO 14040 & ISO 14044) approach.
Pratico et al. (2023) presented a method for comparing pavement technologies from a broader perspective, including LCA-based criteria [ 16 ]. Based on these studies, one question that arises is whether the process of recycling, i.e., carrying out all the necessary waste processing for their incorporation into asphalt mixes (e.g., collection in generation areas, transportation, pelletization, and micronization treatments, etc.) is not more detrimental to the environment than other end-of-life treatments, such as incineration or landfill disposal. With this perspective, it can be concluded that the mere introduction of waste does not guarantee environmentally positive results [ 17 ]. Therefore, there is a need to conduct a Life Cycle Assessment (LCA) that considers the balance of these positive and negative aspects.
In the initial stage, a Life Cycle Assessment (LCA) is conducted considering the Global Warming Potential (GWP) in [kg CO2 eq] of the production of 1 ton of asphalt mix for each one of the studied mixtures. The system boundaries are indicated in Fig. (10). The LCA_Pave software [18] developed by FHWA is utilized, and its inventory is supplemented with bibliographic information. Tables 2 to 5 include the input data. The impact assessment method employed is TRACI 2.1, considering a horizon of 15 years.
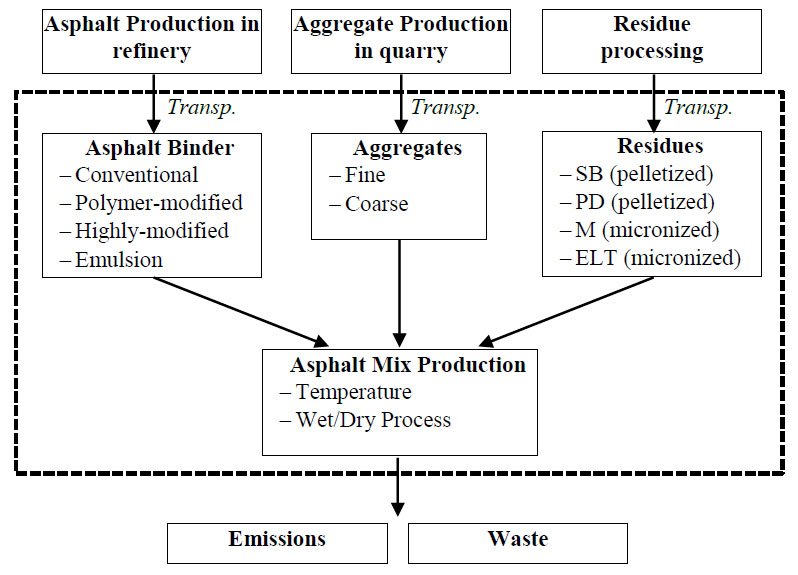
System boundaries for LCA.
Description |
Total mix for h = 10 cm (ton) |
Coarse Aggregate (ton) |
Fine Aggregate (ton) |
Bituminous Binder (ton) |
Residues (ton) |
---|---|---|---|---|---|
SBDW | 778,9 | 283 | 442 | 38,9 | 15,6 |
SBWW | 819,7 | 303 | 474 | 41,0 | 1,2 |
PDDW | 770,5 | 280 | 437 | 38,5 | 15,4 |
PDWW | 812,7 | 301 | 470 | 40,6 | 1,2 |
3M | 783,9 | 283 | 441 | 36,8 | 23,5 |
ELT | 819,4 | 385 | 392 | 38,5 | 3,9 |
WMA | 813,4 | 386 | 386 | 40,7 | 0,0 |
PMA | 827,5 | 391 | 400 | 36,2 | 0,0 |
HIMA | 810,7 | 373 | 395 | 42,9 | 0,0 |
HMA | 797,3 | 295 | 464 | 38,2 | 0,0 |
Note: 1 ton = 1000 kg.
Material | Distance from Production Site to Plant (km) |
---|---|
Coarse Aggregate | 300 |
Fine Aggregate | 300 |
Residues | 80 |
Bitumen | 300 |
Description | 120 °C | 160 °C | 180 °C |
---|---|---|---|
SBDW | - |
![]() |
- |
SBWW | - |
![]() |
- |
PDDW | - |
![]() |
- |
PDWW | - |
![]() |
- |
3M | - |
![]() |
- |
ELT | - |
![]() |
- |
WMA |
![]() |
- | - |
PMA | - | - |
![]() |
HIMA | - | - |
![]() |
HMA | - |
![]() |
- |
Description |
Mixer (hs) |
Milling Machine (for Micronization) (hs) |
---|---|---|
SBWW | 2,9 | 0,0 |
PDWW | 2,9 | 0,0 |
3M | 4,2 | 9,0 |
ELT | 2,9 | 2,0 |
Table 6 shows that WMA generates the lowest emissions per ton produced, followed by the conventional HMA. The other mixes can be considered polymer-modified asphalts, which incur higher impacts due to waste processing, the need to mix the binder with the residues when they are incorporated via a wet process, and the higher mix-manufacturing temperatures.
Description |
GWP (kg CO2 eq/ ton) |
---|---|
SBDW | 69,8 |
SBWW | 71,8 |
PCDW | 69,8 |
PDWW | 71,7 |
3M | 80,1 |
ELT | 72,5 |
WMA | 53,9 |
PMA | 71,0 |
HIMA | 83,5 |
HMA | 68,3 |
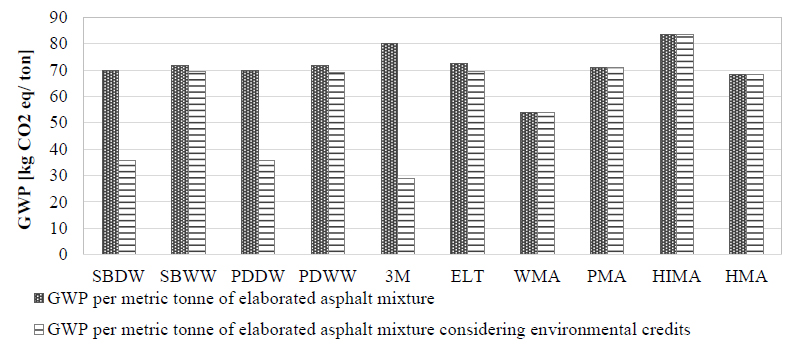
Comparative results of GWP considering the “environmental credits.”
However, it is crucial not to overlook the impact that is avoided when choosing to recycle, i.e., the impact that would occur if waste were given a different end-of-life treatment than recycling [19-21]. This avoided impact is considered an “environmental credit,” and it should be subtracted from the values in Table 5. A comparative graphic is presented in Fig. (11), showing the decrease in GWP values due to this consideration. It is worth noting that the end-of-life treatment alternatives for each type of waste will depend on each region's current legislation and practices.
It is essential to note that this analysis overlooks the performance of the mixes when they are included as part of a structure, meaning the environmental benefits of having an asphalt mix with better mechanical properties and greater durability are not considered. Considering that all base layers have the same thickness (10 cm) but are made of different materials, they will not all have the same life span. Therefore, it is crucial to evaluate the usage stage, which includes road maintenance and rehabilitation processes for a 15-year horizon. It is important to clarify that the initial construction of all pavement alternatives implicates the same environmental impact and therefore, its consideration is not relevant to this comparative analysis.
To delve deeper into this issue, the focus is now turned to the sustainability of structures that incorporate the studied mixes. As an example, the Global Warming Potential (GWP) in [kg CO2 eq] caused by the rehabilitation of 1 km of road for the structure with HMA is evaluated and compared with that of a mix incorporating recycled plastics. The structure with the SBDW mix is chosen for this comparative study.
For both alternatives, rehabilitation involves milling and replacement. The milling depth is 1 cm, followed by a 2 cm sand-asphalt mix layer and then a 3 cm overlay of conventional asphalt mix. It is assumed that the first rehabilitation occurs when the rut depth reaches 12 mm, and the deterioration evolution after rehabilitation follows the same pattern as the initial one. After BackViDe proces-sing, it is concluded that the HMA structure requires two interventions over the 15-year period studied, while the SBDW structure requires only one (Figs. 12 and 13).
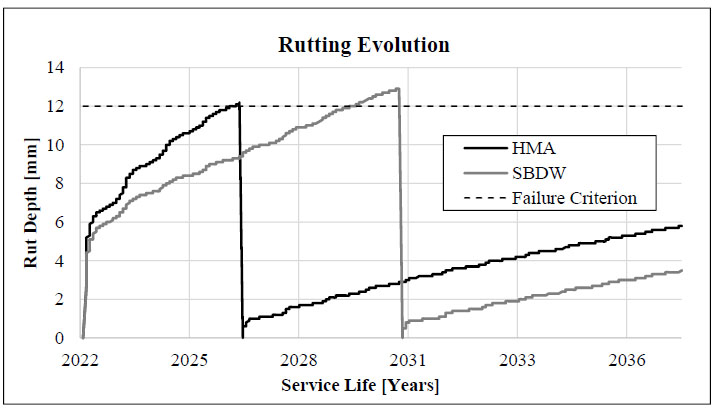
Rutting evolution of different structures.
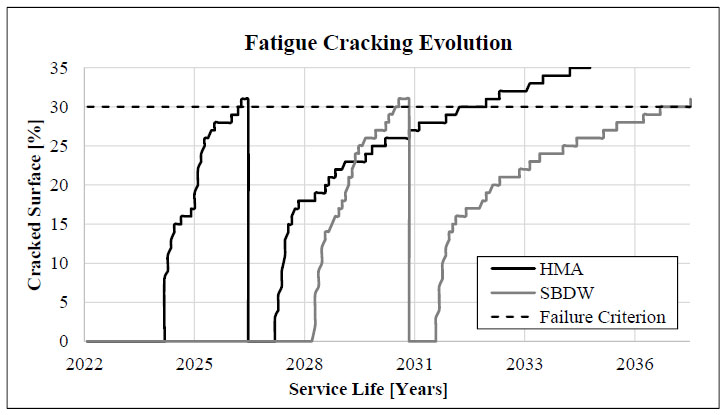
Fatigue cracking evolution of different structures.
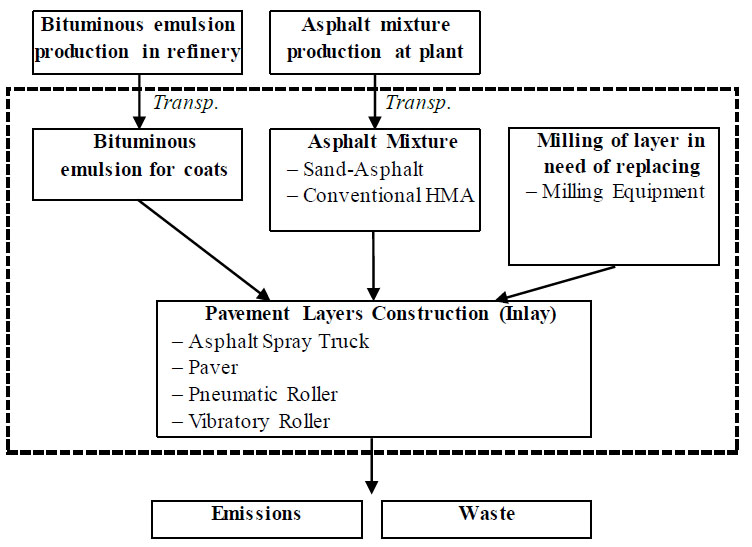
System boundaries.
Fig. (14) illustrates the system boundaries. The hot mix asphalt is transported a distance of 20 km from the production plant to the construction site, and for the bituminous emulsion for coating, 300 km is considered.
After processing the data in LCA_Pave, it is determined that the Global Warming Potential (GWP) associated with the rehabilitation of 1 km of roadway (two lanes of 3.35 m) is 61,534.0 kg CO2 eq. This value corresponds to approximately 77 kg CO2 eq per ton of in situ mix, similar to results obtained by other authors who conducted similar studies [22, 23].
For the structure with CA30, two of these rehabilitations are necessary over the studied 15 years, while for the SBDW alternative, only one is required. Therefore, the HMA structure implies double the impact. The comparative advantage of SBDW lies in the improved mechanical properties that recycled plastic imparts to it, making it a more resistant and durable material. This positively impacts the reduction of the need for mainte-nance and rehabilitation over time.
From another perspective, if one wishes to design a structural package with a specific lifespan, the use of asphalt mixes with improved mechanical properties results in thinner base layers, leading to a reduction of impacts due to the lesser use of resources and energy [24].
3.4. Final Balance of Selection Criteria
It is desirable for construction professionals and public workers alike, to find the most beneficial flexible pavement structure solution. For a certain asphalt mixture to achieve this characteristic, it needs to perform adequately in different aspects: technical, environmental and economical. The weighing of each aspect depends on the priorities that the professional has on every particular task so the decision cannot be final in this study. Fig. (15) shows the performance of each mixture in the studied areas. Clearly, HIMA and ELT mixtures exhibit promising results. SBWW and PDWW have potential as well, regard-less of the chosen weighing.
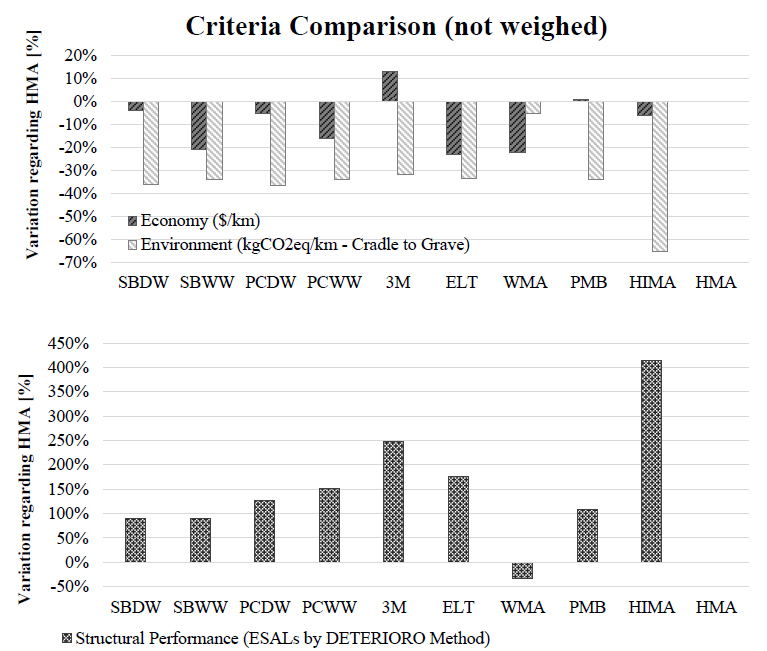
Comparative performance of the studied mixtures.
CONCLUSION
When considering the evolution of asphalt mix design towards a more sustainable approach, there is a need to model the mechanical properties and structural behaviour adequately in order to determine the technical feasibility of these innovative mixes. For this purpose, an analysis is conducted using empirical-mechanistic approaches under initial and incremental conditions. These methods are not yet fully developed in Argentina, so this study contributes to promote their application, proving how important it is to correcly model new mixtures.
The lifespan of flexible pavement differs when evaluated under initial conditions or incremental ones. In incremental condition analysis, failure due to subgrade deformation prevails, while in initial conditions, failure occurs due to fatigue cracking of the bound base layer. The VIDA module (Initial Conditions) does not consider cracking, deterio-ration, or the modulus variation with temperature. There-fore, it asserts that bound layers consistently protect the underlying layers, leading to fatigue failure. Rutting failure does not occur due to the high thickness of unbound layers.
The DETERIORO module (Incremental Conditions) does consider the variation of the dynamic modulus with temperature, which in most cases results in a more favorable scenario, extending the structure´s lifespan. According to this model, failure occurs due to the accumulation of vertical deformations caused by the weakening of surface layers over time. Although rutting failure is reached, it occurs at a higher number of ESALs than indicated by VIDA. Therefore, it is concluded that, in reality, failure occurs due to rutting, as asphalt layers last longer than suggested by VIDA. This demonstrates why a more sophisticated approach than the one currently used in Argentina is necessary, emphasizing the need to introduce newer test methods to assess the mechanical properties of the mixtures involved. The recommendation of employing mechanistic-empirical design methods is key and applicable to all countries, considering that each one has a diverse, productive matrix and, therefore, different residues susceptible to being incorporated in asphalt mixtures.
It is also observed that asphalt mixes SBDW, SBWW, PDDW, PDWW, 3M, and ELT tend to exhibit behaviour similar to PMA. Structures with these mixes demonstrate better fatigue performance than the control mix. This is attributed to the well-known effects of incorporating polymers into asphalt mixes, significantly improving mechanical properties and sensitivity under temperature variations. The addition of recycled materials in the dry-way results in stiffer mixes in comparison to the ones obtained via a wet process. The WMA tends to behave similarly to the HMA, showing lower dynamic moduli and higher thermal susceptibility. The HIMA mixture has better rheological characteristics due to its high percentage of incorporated polymers and asphalt content, resulting in significantly longer service lives compared to the other mixtures studied. The conclusion that ensues is that all mixtures studied are technically feasible.
From the Life Cycle Assessment (LCA), it is derived that achieving asphalt mixtures with improved mechanical properties is crucial for reducing layer thicknesses or obtaining greater durability for a certain thickness, leading to lower rehabilitation needs. The benefits of reducing energy consumption in the mixture production stage and incorporating waste from other processes in relevant quantities and with minimal processing are also highlighted. Finally, it is added that transportation accounts for an average of 60% of the environmental impact. Therefore, determining the most adequate, environmentally speaking, mixture depends heavily on the transportation distances for each specific project, making the use of local materials essential.
The application of an LCA approach is essential to evaluate the environmental aspect of every major civil engineering project, particularly when different alternatives are involved. Argentina is far behind when it comes to this matter, so this study will hopefully help broaden the mind and raise awareness of this issue.
It can be concluded that sustainability in civil engineering is a matter of utmost importance to ensure long-term viability and to emphasize the role of sustainable practices in mitigating environmental impact.
LIST OF ABBREVIATIONS
SB | Silo-bags |
LPDE | Low-Density Polyethylene |
PD | Phytosanitary Drums |
HDPE | High-Density Polyethylene |
ELTs | End of Life Tires |
LCA | Life Cycle Assessment |